Casting aside CRISPR scissors and making a point with base editors
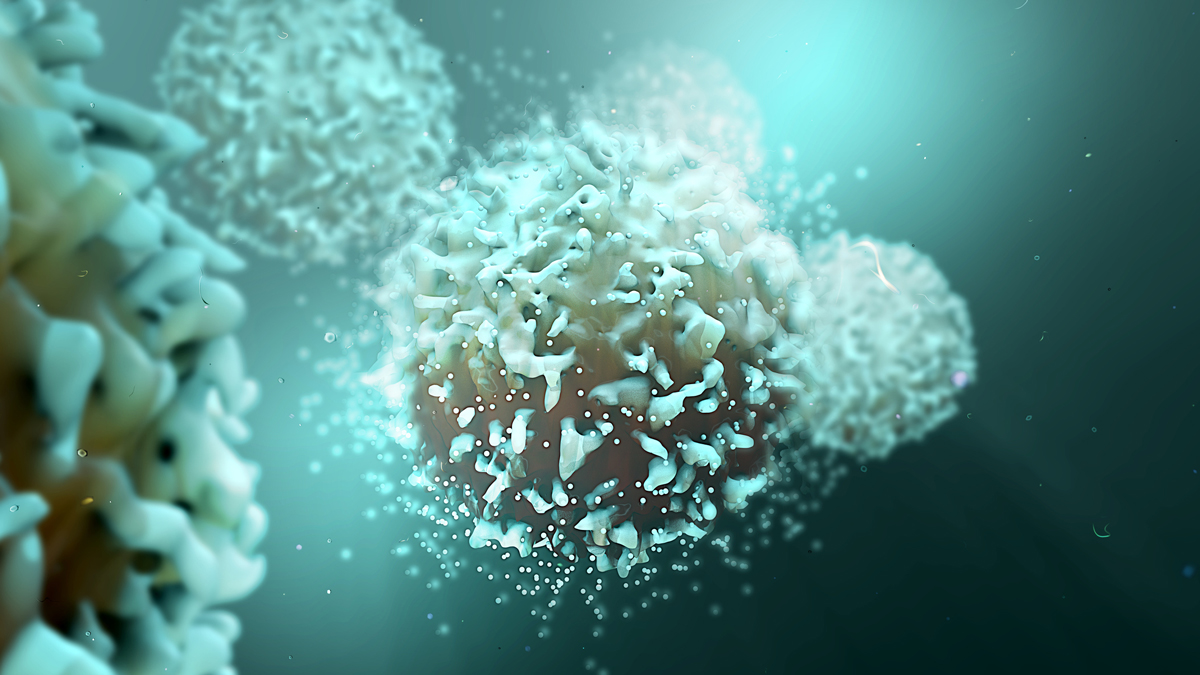
Dr Jennifer Harbottle, senior scientist in the R&D Base Editing team of PerkinElmer’s Horizon Discovery business, looks at progress made in the realms of biotechnology and next-generation diagnostics, vaccines and therapeutics, including the application of CRISPR-Cas9 gene editing in developing and refining cell therapies.
While achieving the Nobel Prize spotlight would have been enough to impress, CRISPR-Cas9 gene editing is part of a growing list of technologies granted Investigational New Drug (IND) applications with early data from clinical trials supporting its safe use in edited cells re-introduced into a patient.
However, caveats surrounding the stochastic off-target outcomes of cleaving both strands of the DNA helix to elicit a gene modification remain a source of concern. The emergence of novel base editing technologies that do not generate double-strand breaks (DSBs) offer a promising and safer alternative to fine-tune the next generation of immune cell therapies, especially as our need to perform complex multi-gene alterations increases.
Improving on impressive first steps
Kymriah and Yescarta were approved by the US Food and Drug Administration in 2017 for the treatment of refractory or relapsed B-cell haematological malignancies. These treatments are examples of patient-specific, engineered chimeric antigen receptor (CAR)-T cell therapies that harness the immune system to target and eliminate cancer cells.
However, despite huge success with high early response rates, durable disease remission remains impressive but limited in terms of patient numbers. Also, to date this treatment strategy has not translated well to solid tumours, where engineered CAR-T cells face challenging conditions including a notoriously immunosuppressive tumour microenvironment. Gene editing has been put forward to overcome these barriers.
A recent report indicates that autologous T cells that carry multiple CRISPR-Cas9-mediated genetic modifications designed to improve persistence and efficacy can be safely administered to patients with refractory cancer, providing an important step forward in the use of CRISPR in immune cell therapies.
However, the number of CAR-T cells with all four genes correctly targeted was low. This can be partly attributed to the reagents and delivery modalities that were available at the time of the trial start in 2016, leading to low editing efficiency at the target loci. While it is expected that more advanced reagents and techniques will improve the levels of on-target editing, it is also becoming increasingly clear that simultaneous alteration of more target genes will be required for successful proliferation, persistence, and efficacy of CAR-T cell therapies, particularly against refractory solid tumours.
Another area for potential improvement is the source of immune cells. The paradigm of patient-derived autologous CAR-T cell production hinders controllable or scalable manufacturing and on-time treatment for patients. Thus, the availability of universal, “off-the-shelf” engineered T cells presents an attractive alternative. Such allogeneic products, pre-manufactured from healthy donors, would bypass the issues of insufficient, variable, or poor-quality source material from patients, overcome manufacturing delays, and ultimately ease the commercialisation of CAR therapies and usher in a more equitable deployment of CAR-T cell therapies.
However, further genetic tweaks are required to accord allogeneic CAR cells a “stealth mode”, to avoid graft-versus-host-disease toxicity but also to escape host-mediated rejection. Additional alterations are also needed to spare engineered CAR-T cells in patients treated with lymphodepleting agents, and/or to modify genes involved in the occurrence of cytokine storms or neurological toxicity, common side effects of CAR-T cell therapies.
Long story short, simultaneous editing of a large array of genetic loci will be required to enhance the efficacy and persistence of engineered CAR-T cell therapies, to expand the range of targetable malignancies, and to contribute towards the development of safe allogeneic products. As more gene candidates are identified, the potential off-target outcomes of DSB-based editing increase substantially.
Will double-strand breaks burst the bubble of next-generation immune cell therapies?
Safety is paramount in the successful translation of gene editing technologies to the clinic. Traditional CRISPR-Cas9 technology relies on generating a DSB in the DNA to facilitate a genetic modification. Despite being an effective strategy to disrupt gene transcription and downstream protein expression, the introduction of random insertions and deletions (indels) at the site of the DSB can result in inadvertent alterations and pathogenic lesions at the target locus and elsewhere in the genome.
Concerted effort has been put into mitigating the occurrence of off-target events, but while it is possible to predict and map the risks of unwanted edits for one or two target genes, it is far more complex to assess the outcome for larger numbers of genes. Additionally, although rational guide RNA (gRNA) design, transient delivery modalities (mRNA, ribonucleoprotein) and the use of high-fidelity nuclease variants will all limit DNA cleavage at unintended sites in the genome, these strategies do not address the inherent risks associated with on-target DNA mutagenesis through the introduction of a DSB.
Multiple break points in the genome might not only trigger a DNA damage response and cell death but could also generate translocations and chromosomal rearrangements. The occurrence of such genomic aberrations increases in the context of multiplex editing where concurrent DSBs yield multiple free DNA ends that are amenable to repair and annealing in a variety of different configurations.
The consequences of this genetic scrambling are largely unknown and unpredictable but could lead to the production of oncogenic fusion genes or disruption of essential genes or cell function. This is not an ideal scenario for clinical application. Iterative introduction of CRISPR-Cas9-mediated edits would potentially raise the safety profile of engineered T cells, but this would be arduous, time-consuming, expensive, and inefficient.
A better approach to multi-gene editing would be one that does not generate DSBs; this is where CRISPR-derived base editing offers a distinct advantage.
Base editing to get cell therapies on point
While a traditional CRISPR-Cas9 platform cleaves both strands of the DNA helix to elicit indel formation, via an error-prone non-homologous end joining repair pathway, base editors nick a single strand of DNA to trigger high-fidelity mismatch repair and nucleotide alteration mediated by a deaminase, thereby largely preventing indels, translocations or other unwanted chromosomal rearrangements.
Avoiding the risks associated with CRISPR-Cas9-mediated DSBs and indel formation for the purpose of genetic knockout, base editors allow for controllable and effective gene inactivation via the precise introduction of stop codons in key protein-coding regions, or through the disruption of highly conserved splice sites.
In light of the growing number of edits required to enhance the scope, efficacy and persistence of engineered immune cell therapies, base editing is a strong contender for efficient multi-gene targeting with substantially reduced risk of causing inadvertent damage in the genome.
Base editors are formed of two main components: a catalytically impaired Cas enzyme to introduce a nick into the DNA, and a deaminase effector protein that chemically alters a target nucleotide within a narrow editing window defined by a programmable gRNA. Although there have been many iterations of base editors since their inception in 2016, cytidine base editors (CBE) and adenine base editors (ABE) form the two main categories that mediate efficient C-to-T and A-to-G base changes, respectively.
Base editing technology, first reported and published from Harvard University, is a true demonstration of “precision chemical surgery”. Other base editing platforms, such as that from Rutgers University, have also been developed, and it is at breakneck speed that base editing technology begins to enter the therapeutic realm.
Barely five years since the fundamental descriptions of the first base editors, both the CBE and ABE platforms have been used in pre-clinical studies to mediate simultaneous editing of multiple genes in engineered T cells. The results are encouraging; modified cells show high levels of editing across all target loci and cells retain functionality, while showing no evidence of unwanted off-target events or translocations. Impressively, the past year has highlighted exciting examples of gene correction using base editing technology, including pre-clinical correction of gene mutations underlying progeria, glycogen storage disease, alpha-1 antitrypsin deficiency and sickle cell anaemia. The rapid progress and promising outcomes to date make base editing a strong contender in this exciting and fast-paced era of precision medicine.
About the author
Dr Jennifer Harbottle is a senior scientist in the R&D Base Editing team of PerkinElmer’s Horizon Discovery business. Since joining the Company in May 2019, her work has contributed towards the development and commercialisation of the base editing platform, including a focus on application of the novel technology in immunotherapies and development of strategies to assess the precision and safety profile of selected agents. Jennifer obtained her PhD in Cell Biology at the University of Aberdeen and was first intrigued and enticed by base editing in 2018 during an R&D project at Oxford Genetics (now OXGENE).